In 2016, NACE International released its report on global corrosion, “International Measures of Prevention, Application and Economics of Corrosion Technology (IMPACT)”. Put together over two years, the study came to some staggering conclusions about the price of corrosion, and the room for improvement when it comes to preventing it.
IMPACT estimated the annual global cost of corrosion to be $2.5 trillion: equivalent to almost 3.5 per cent of global GDP, and only a few hundred billion dollars short of the UK’s entire annual domestic output.
By implementing best-practice corrosion protection, the study said, this cost could be reduced by up to a third, or between $375 and $875 billion. With such huge amounts of money at stake, it’s little wonder that companies around the world are investing so heavily in developing more effective corrosion protection strategies.
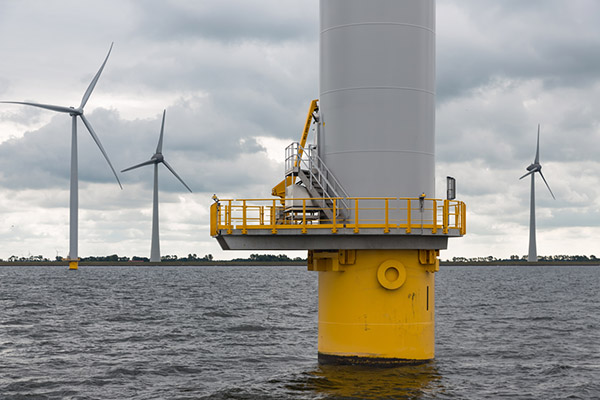
What is corrosion?
Corrosion is a complex phenomenon that can occur in a variety of ways, but essentially, it is the dissolution of metal due to a chemical reaction with its environment. The metal loses electrons through an electrolyte, such as seawater, as it breaks down at an atomic level.
Some metals corrode more readily than others, but under the right conditions, even the most chemically inert metals can corrode. This even applies to the noble metals such as gold, silver and platinum, and can be seen in tarnish, in which corrosion creates a dull outer layer on objects made from silver.
Corrosion can be a problem in air, but it is a far more significant issue in seawater. Here the process is initiated by the presence of oxygen and greatly exacerbated by the prevalence of chloride, which makes the seawater an excellent conductor.
Taking one for the team: cathodic protection
At sea, the primary form of corrosion mitigation is cathodic protection. Typically this involves the use of a galvanic, or “sacrificial” anode: a piece of metal connected to the structure requiring protection, and made from a more electrochemically active material.
Connected together, the structure under protection and the sacrificial piece of metal form an electrochemical cell. The structure serves as the cathode, while the sacrificial metal serves as the anode.
Because in corrosion it is the anode from which electrons are lost, the structure – the cathode – is thereby protected from deterioration. The galvanic anode corrodes in its place, disintegrating in the process, and sacrificing itself to protect the structure.
These galvanic anodes are made from aluminium, zinc or magnesium, and usually take the form of plates or blocks. By definition they require periodic replacement, and with the costs of doing so not insignificant, more cost effective means of corrosion protection remain in high demand.
Cathodic protection systems are often used together with a protective barrier coating on the main structure, which reduces its exposure to the seawater and effectively reduces the size of the electrochemical cell’s cathode. A smaller cathode allows a smaller sacrificial anode, which means less material and reduced cost.
A different approach: combining the coating and the anode
With barrier coatings providing one aspect of corrosion mitigation, and cathodic protection another, one potential way to improve the two approaches’ cost-effectiveness is to combine them into a single function.
This is where thermally sprayed aluminium (TSA) comes in. In this process, microscopic droplets of molten aluminium are fired at very high speed onto a substrate – such as a steel wind turbine foundation pile – where they bond with the underlying structure, and with each other, creating a high-integrity barrier that doubles up as a “distributed” anode should any of the underlying steel become exposed to the seawater.
Aluminium is a perfect material to serve as a protective barrier, as it possesses the extraordinary ability to repair itself. As soon as it corrodes, an impermeable oxide layer forms on its surface, protecting the underlying metal from further corrosion. Should the metal beneath become exposed, an oxide layer will quickly form to plug the gap.
A TSA barrier may remain effective for up to 50 years at sea, with minimal maintenance costs. There are big savings to be made here: one company’s decision to replace its conventional paint and sacrificial anode corrosion protection system with TSA for two offshore turbine foundation piles is expected to lead to savings of approximately 30 per cent.
Built to withstand the ocean swell
Corrosion isn’t the only destructive power marine structures must resist: they must also endure the relentless assault of the movement of the sea. With the typical wave frequency being approximately once every five seconds, over the course of 20 years a structure at sea must withstand more than 126 million cycles. Even if the loads involved are relatively low, that represents a tremendous amount of fatigue damage. Structures exposed to such environments experience fatigue – material deterioration in response to repeated loads. Marine structures are designed and built with high fatigue resistance in mind, and design codes such as BS EN ISO 19902 and DNVGL RP-C203 stipulate in detail the standards they must meet.
These codes take into account the impact of aqueous corrosion: structures exposed to seawater are subjected to an environmental reduction factor of three compared to structures in air. This means, under free corrosion conditions, a structure would achieve a fatigue design life in seawater just one third of the duration it would have in air.
The next step: proving the fatigue performance of TSA-coated parts
In the interests of safety, design standards understandably tend towards conservatism. However, with the emergence of more effective corrosion protection techniques, there may be cause to relax some of these design requirements.
Much information already exists on fatigue performance. Likewise, the effect of TSA on corrosion has been widely investigated. However, the fatigue performance of TSA-coated welded joints has not been properly explored, and doing so could yield scientific data that would make the savings inherent in using TSA for corrosion protection even more significant.
Such data could underpin revisions to design codes and support TSA gaining international acceptance as an alternative to conventional corrosion protection systems, leading to lower material usage, lower maintenance requirements, and lower costs.
For more information, please contact us.